1. Introduction
Hundreds of individual organic compounds have been identified
in the organic atmospheric aerosol so far (e.g. Saxena and Hildemann,
1996), however, together they constitute less than 10% of the
organic carbon (OC) of urban and rural aerosol (e.g. Rogge et
al., 1993a; Puxbaum et al., 2000). The main analytical method
used so far for separating and identifying organic individual
species was Gas-Chromatography coupled to Mass Spectrometry
(GC/MS). While aerosol extracts of non polar and weakly polar
species were directly accessible to GC/MS analysis, for polar
species such as organic acids, carbonyls, and multi functional
compounds various derivatisation reactions had to be employed
to increase the range of species to be identified. One of the
recent results of the use of new derivatizing reagents was the
observation of levoglucosan and related anhydrosugars in aerosol
samples and their use as tracers for biomass combustion (Simoneit
et al., 1999); as well as of novel di- and tricarboxylic acids
in fine tropical aerosols (Zdrahal et al., 2001). Very recently
Claeys et al. (2004) identified tetrols as major oxidation products
of isoprene in aerosols from the Amazonian region.
The
analysis of extractable organic fractions so far concentrated
on molecules accessible to Gas Chromatography – with carbon–atoms
generally less than 40. From considerations of the solubility,
the behavior in the thermoanalytical methods as well as from
observations with microscopical techniques we conclude that
a large and until recently unaccounted fraction of the continental
organic aerosol consists of polymeric or oligomeric substances.
Rogge
et al. (1993a) and Zappoli et al. (1999) have shown, that a
considerable part of the organic aerosol is not soluble in water
and organic solvents, which points to larger molecular sizes
of the insoluble compounds. Matthias-Maser and Jaenicke (1995)
have demonstrated that up to 40% of the number of particles
> 0.2 µm AD over a continental site were considered of "biogenic
origin". The high contribution of biogenic material to
the particle number concentration points to biopolymers as a
main source for the insoluble organic constituents in the atmospheric
aerosol. Bauer et al. (2002a) introduced a quantification method
for calculating the contribution of spores to OC in aerosols
based on spore counts, and found considerable amounts of carbon
from spores in background aerosol in Austria (Bauer et al. 2002b).
But, to make the story still more complicated, the main constituents
of the organic aerosol according to current knowledge are the
“Humic Like Substances” (HULIS), occurring in the aerosol as
water soluble as well as water insoluble fractions (Havers et
al., 1998). HULIS are present ubiquitously in continental aerosol
samples at concentrations (HULIS-carbon) from 7-24 % of the
OC (Havers et al., 1998; Zappoli et al., 1999; Facchini et al.,
1999). HULIS are definitely macromolecular substances, possibly
with manifold origin (e.g. from biomass burning – Facchini et
al., 1999; or from secondary reactions in the atmosphere – Jang
and Kamens, 2001; Gelencser et al., 2002 & 2003; Limbeck
et al., 2003; Iinuma et al.,
2004, Kalberer et al., 2004).
Here
we compile available data to investigate which species in the
organic aerosol contribute significantly to the so far “unaccounted organic carbon”,
and which part is still unaccounted..
2. Unexplained and
Unresolved Organic Mass
2.1. Indications from
Group-Specific Methods
Solubility
In
different reports it was made clear that a larger fraction (e.g.
up to 50%) of the organic carbon in urban aerosol was not soluble
in solvents of different polarities (e.g. Rogge et al. 1993°; Zappoli et al. 1999).
Insolubility in water and organic solvents is a property observed
widely in natural systems, in particular cell walls of plant
and animals. Such bio-polymeric material is based on cellulose,
hemicelluloses and lignin in plants, and on different forms
of proteins in animals. Also anthropogenic polymeric materials
are generally insoluble: different types of plastics, fibers
and synthetic rubber. The
high fraction of insoluble material in continental aerosols
is indicative for the polymeric state of the compounds, potentially
of natural as well as anthropogenic origin.
According
to Weissenbök et al. (2000) 70% of the insoluble filterable
particulate carbon from snow collected in Austria at 3100 m
elevation accounted of “modern” carbon, which is taken as evidence,
that biogenic material dominates the insoluble aerosol fraction
– even in the mid troposphere.
Microscopical Detection of Bio-Particles
The
use of microscopical techniques for identifying the nature of
individual particles dates back a long time. A large collection
of individual particles identified with light microscopy as
well as electron microscopy was edited by McCrone and Delly
1973 (“Particle Atlas”).
Matthias-Maser
and Jaenicke (1995) introduced a combination of light and electron
microscopic techniques allowing a quantitative assay of the
fraction of “bio-particles” related the total number of particles
in an air sample. Their method for the larger particles is based
on staining the protein-containing particles and observing blue
colored vs. black or non-colored particles. For the smaller
particles REM investigation, combined with EDS spectra was applied.
Bio particles were identified according to their shape and content
of bio-tracer elements (e.g. Phosphorus and Potassium).
Bauer
et al. (2002a) investigated the carbon content of different
species of airborne spores and used these numbers for determining
the contribution of bacteria and fungal spores to the organic carbon
content of cloud water, precipitation and aerosols. Fungal
spores were found in the size fraction of 2.15-10 µm of organic
background aerosol at a mountain site forming on the average
6 % of the organic carbon (OC) of the “coarse” size fraction
(Bauer et al., 2002b).
Pollen
was considered of less importance for PM10 or PM2.5 aerosol
size fractions. However, Schäppi et al. (1997) demonstrated,
that some types of pollen grains expel upon influence of rain
water much smaller particles, in the case of birch pollen of
allergenic property. Thus, pollen may also be a source of fine
particles.
In
a larger cooperative project taking place during the non-burning
season in Amazonia a range of techniques was applied to quantify
the contribution of natural sources to OC, based on microscopic
and advanced chromatographic techniques (Blaszo et al., 2003; Graham
et al., 2003).
Thermographic Techniques
Analysts
applying thermographic techniques for determining OC were for
long aware, that a “refractory” organic carbon fraction is omni
present in atmospheric aerosols. Puxbaum (1979) described cracking
of biopolymers as source of overlapping peaks with the black
carbon peak. He investigated the thermal behavior of dried leaves,
wood, pollen, natural rubber and lignin. All these substances
formed double peaks during linear temperature programmed heating
in oxygen, with partial overlap of the black carbon peak. The
group of compounds showing the double peaks was referred to
as organic debris. Also Ellis and Novakov, 1982 identified in
thermograms of rural aerosol samples poorly resolved peaks (marked
with a, b,
g, d),
corresponding to volatilization and/or oxidation of carbon species
of increasing thermal/oxidation stability. After normalization
to the source thermograms the excess peaks b
and g— were hypothesized to
be high molecular weight polymeric material, however, suggested
to be a first-order measure of secondary organic carbon.
|
|
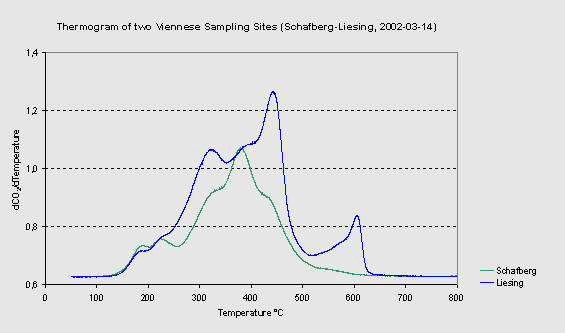 |
Figure 1: Thermograms (linear
heating in O2) of two aerosol PM10 samples from an
urban industrialized (Liesing) and a suburban (Schafberg) site.
The highest peak at 440°C is BC, the last peak at 600°C is from
carbonates. The “refractory OC” peak at 380°C is present in
the background air and does not increase from urban activities.
back
to top
Chromatographic Techniques
Coupling
of Liquid Chromatographic set ups with Mass Spectrometers
applying Electrospray Ionisation (“ESI-MS”) allowed to determine
the range of the molecular weights of organic compounds from
aerosol extracts assigned to the HULIS-Fraction (e.g. Kiss et
al., 2003). Similarly, formation of polymers from smog chamber
experiments was demonstrated using capillary electrophoresis
– ESI-MS (Iinuma et al., 2004) or laser desorption ionization
mass spectroscopy (Kalberer et al., 2004).
3. Classification of Atmospheric
“Bio-Aerosols”
“Bio-Aerosols”
in the atmosphere may be viable or “dead”, detritus or debris
of living matter, plants or animals. Such particles we will
classify as “primary biogenic” particles. However, more recently,
results from laboratory study indicate, that aerosol might also
form on secondary pathways in the atmosphere from biogenic emissions.
Table 1: Classification
of Bio-Particles or related aerosol constituents
PRIMARY |
|
|
VIABLE |
Bacteria
Fungal
Spores
Algea
Pollen |
Sattler et al. 2001
Bauer et al. 2002a, b
Schäppi et al. 1997 |
DEBRIS/DETRITUS |
Cellulose
Lignin
Amino Acids |
Kunit and Puxbaum 1996
Blazso et al. 2003
Zhang and Anastasio 2003 |
“TRACER” |
Hydrocarbons
Fatty
Alcohols
Fatty
Acids
Etc. |
Simoneit
1980 |
SECONDARY |
|
|
“SMALLER
MOLECULES”
(<
300 Da) |
Terpene-Oxidation
Prod.1)
Tetrols (Isoprene-Oxidation Products) |
Kavouras
et al. 1998
Claeys et al. 2004 |
“MACROMOLECULES”
(>
300 Da) |
HULIS2) |
Havers
et al. 1998 |
1) Aerosol formation via absorption in
pre-existing organic aerosol
2) HULIS are not exclusively of biogenic origin
4.
Humic-Like Substances (“HULIS”) in the Atmosphere
OBSERVATIONAL
STUDIES OF PHYSICAL AND CHEMICAL PROPERTIES OF HULIS
In mass closure studies on organic aerosol “humic-like substances
(HULIS)”, or “organic macromolecules”, or “polycarboxylic acids”,
are generally claimed to resolve a large fraction of unexplained
mass of particulate organic carbon. Strictly speaking, however,
HULIS are not and can never be speciated, their presence can
only be inferred from various bulk aerosol measurements. Although
by now there is conclusive evidence that such compounds are
ubiquitous in the water-soluble organic aerosol, their quantitative
determination is still subject to great conceptual and experimental
uncertainties.
Humic acids were first reported in aeolian dust by Simoneit
(1977), and these findings were confirmed by Simoneit and Mazurek
(1982) who found that humic acids constituted a major fraction
of rural aerosol. Based on observed H/C ratio and d13C
values, a mixed origin from soil and lacustrine mud was inferred
by the authors. Therefore the occurence of HULIS in soil-derived
aerosol seemed to be well-understood.
At about the same time, another line of research was started
which later turned out to be more relevant to HULIS as we see
it now. These studies were the very first to establish the presence
of polymeric matter in fine aerosol and postulated its secondary
origin. In the thermograms of rural aerosol samples poorly resolved
peaks were distinguished by Puxbaum 1979, and postulated to
origin from biogenic polymeric substances. Similarly, Ellis
and Novakov (1982) observed such peaks, marked them with a,
b, g,
d, corresponding to increasing
thermal/oxidation stability, respectively. After normalization
to the source thermograms the excess peaks b
and g— were suggested to be
a first-order measure of secondary organic carbon— and were
hypothesized to be of high molecular weight polymeric material,
judged solely on the basis of their thermal/optical properties.
This idea was far too premature at that time to gain widespread
recognition. It took another 15 years for the first field observations
to rediscover atmospheric polymers (Havers et al., 1998; Zappoli et al.,
1999).
Combination of the thermal technique with water extraction
revealed additional features of the bulk organic matter. For
example, about half of the refractory aerosol component appeared
to be soluble in water (Gelencsér et al., 2000a). The thermal properties
of this refractory carbon differed markedly from those of the
coarse aerosol, but seemed to resemble those of a reference
humic acid on pre-baked quartz filters.
The bulk characterization of organic carbon, in particular
of its water-soluble fraction led to the surprising conclusion
that almost all observed properties resembled closely those
of natural humic substances. The first observations of this
kind by UV-VIS spectrophotometry and proton nuclear magnetic
resonance spectrometry (HNMR) were made on urban particulate
matter (Havers et al.,
1998). These authors were the first to introduce the term “humic-like
substances”, HULIS, which has become widely accepted in the
literature.
At about the same time, a comprehensive study was published
on the bulk properties of water-soluble organic matter in aerosol
from a polluted, rural and marine environment (Zappoli et
al., 1999). Their major finding was that WSOC made up a
significant fraction of fine aerosol carbon, and its detailed
analytical characterization revealed a stunning resemblance
to a reference humic acid. In spite of the high degree of similarity,
the authors refrained from using the term “humic-like” for this
major class of compounds. Instead, they termed this fraction
“macromolecular”, though none of their analytical methods yielded
direct evidence that these species were indeed of high molecular
weight. They suggested biomass burning to be most likely source,
and postulated direct condensation of high-molecular weight
burning products as a possible formation mechanism. Their hypothesis
assigned primary anthropogenic origin to this compound class,
and was able to account for its observed abundance in the fine
particle size range.
These pioneering works induced further studies on the occurrence
and properties of HULIS in atmospheric aerosol. New analytical
techniques were used to reveal the properties of the bulk WSOC,
and to compare them to those of natural humic matter. It was
shown that the electrochemical properties and metal-complexing
ability of bulk organic matter in polluted fog water were nearly
the same as those of a reference humic acid (Gelencsér et al., 2000b). Other studies applied various
separation methods to characterize such compounds, most of which
were based on their acid-base properties. These efforts unambiguously
proved that a traditional speciation will not be possible: the
chromatograms or electropherograms showed a few poorly resolved
broad peaks and/or an unresolved “hump”. Capillary zone electrophoresis
of polluted fog water and aqueous extract of rural fine aerosol
suggested a broad distribution of charge-to-size ratios of HULIS
(Krivácsy et al.,
2000). The observed pH-dependence implied that most acidic groups
were found to be weaker acids than acetic acid.
Decesari et al.
separated the WSOC of the fine aerosol collected at a polluted
site and the organic fraction was divided into there generic
classes by preparative ion-exchange chromatography (2000). These
were neutral/basic compounds, mono- and dicarboxylic acids,
and polycarboxylic acids (with at least 3 negative charges per
molecule). They determined the chemical structure of these broad
compound classes by 1HNMR spectrometry. The spectra
of the neutral/basic compounds revealed the presence of mainly
hydroxylated/alkoxylated aliphatic species, with indications
for the presence of polyols. Mono- and dicarboxylic acids were
shown to be predominantly aliphatic carboxylic acids and hydroxy
carboxylic acids, whereas polycarboxylic acids had a more pronounced
unsaturated character, with an aromatic core having aliphatic
chains with −COOH, −CH2OH, −COCH3
or −CH3 terminal groups. The observed features
of the polyacidic compounds closely resembled those of terrestrial
and aquatic humic matter. In general, the polycarboxylic acids
were the most abundant class of WSOC throughout the year, except
in summer, when mono- and diacids were predominant (Decesari
et al., 2001). It
should be noted that a large fration of the WSOC was UV-absorbing,
and the specific UV-absorptivity was highest for the class of
polycarboxylic acids. On the basis of group separation and HNMR
measurements of the WSOC fraction, a representative mixture
of individual compounds was suggested to simulate the physical
and chemical properties of aerosol WSOC in model calculations
(Fuzzi et al., 2001).
The selection of the model compounds should be regarded as a
conceptual approach which would serve as a basis for further
research on organic aerosol.
Varga et al. developed another preparative-scale separation method for the
isolation of HULIS from the aqueous extracts of aerosol (2001).
They carefully optimized their method to isolate the fraction
of WSOC that retained the key spectral properties also characteristic
of reference humic and fulvic acids. Their separation was based
on molecular interactions with the non-dissociated species,
and is therefore conceptually different from the method based
on the separation of ions, since polycarboxylic acids can be
separated both in their ionic and molecular forms. Therefore
both methods are believed to target broadly the same generic
class of compounds, i.e. the terms “polycarboxylic acids” and
“HULIS” possibly refer largely to the same fraction of organic
aerosol. Since, however, there has been no intercomparison between
these methods this statement is merely based on an assumption
derived from the observed chemical properties of the isolated
compounds, as well as on the fundamental principles of the separation.
There is, however, an important conceptual
difference between the two methods. The method by Varga et al. (2001) allows the isolation of HULIS
from inorganic compounds too, which is not possible in method
by Decesari et al.
(2000). This fact allows analytical determinations to be performed
which otherwise would not be feasible in the presence of interfering
inorganic species. For example, the elemental composition of
HULIS isolated from rural fine aerosol was found to be remarkably
constant throughout the year, corresponding to an average molar
ratio of C:H:N:O of 24:34:1:14 (Kiss et
al., 2002).
An important step towards the understanding
of the origin of HULIS in rural aerosol collected in summer
was the determination of their molecular weight distribution
by ultrafiltration, liquid chromatography-atmospheric pressure
ionization mass spectrometry, and vapor pressure osmometry (Kiss
et al., 2003). The
most interesting finding of this study was that virtually all
WSOC passed through an ultrafiltration membrane having a 500
Da nominal molecular weight cut-off. The isolated HULIS—which
made up of more than half of WSOC by mass—was further characterized
to determine their ion mass distribution which was found to
be continuous between about 100 and 500 Dalton, with maxima
in the range of 200-300 Da. These conclusions were confirmed
by vapor pressure osmometry which provided direct estimates
for the average MW of HULIS. The average molecular weight was
found to be markedly lower than those of reference aquatic humic
and fulvic acids under the same conditions. These observations
are among the very few that pointed to important differences
between HULIS and natural humic substances, and imply distinct
mechanisms of their formation.
Yu et al. have recently evaluated the mass size distributions
of WSOC in marine and continental aerosol (Yu et
al., 2004). Regardless of their origin, WSOC in aerosol
exhibited a bimodal size distribution, with a dominant fine
mode and a minor coarse mode having mass mean aerodynamic diameters
of 0.7± 0.1 and 4.0 ± 0.3 mm, respectively. The mass
in the fine mode ranged from two-thirds to four-fifths of that
of the total WSOC. Both modes were further deconvoluted to low,
medium, and high molecular weight polar compounds based on their
thermal evolution features. While the low MW species had a bimodal
distribution with a dominant coarse mode, the medium and high
MW compounds exhibited a single peak in the droplet mode. This
was interpreted as evidence that these latter species—which
might also be humic-like substances—likely form during cloud-processing
of aerosol. This finding would support the possible formation
of HULIS in cloud processes (Gelencsér et al., 2003).
In fact, very few analytical techniques
are capable of providing chemical information directly on the
carbonaceous component of the bulk aerosol collected on filter
substrates or impactor plates. One of these methods is pyrolysis-gas
chromatography-mass spectrometry which allows organic structure
elucidation directly from aerosol filters. Taking into account
the fact that HULIS contain functional groups (e.g. carboxylates)
which yield non-specific thermal decomposition products (e.g.
carbon dioxide) upon conventional analytical pyrolysis, in its
very first application in aerosol chemistry a derivatization
technique was introduced (Gelencsér et
al., 2000c). The thermally assisted hydrolysis-methylation
allowed labile functional groups to be converted into their
respective esters, thus preventing decarboxylation upon pyrolysis
and yielding more specific pyrolysis products. The analysis of rural fine aerosol by this method revealed
overall structural similarities to those of natural humic substances.
The predominant pyrolysis degradation products both in aerosol
and terrestrial humic acids were n-alkanoic acids, a,w-dicarboxylic acids (in the carbon number range of C4–C9),
and benzenedicarboxylic acids. The apparent structural similarity
to terrestrial humic substances made the authors suggest the
term “atmospheric humic matter” in place of HULIS. The rationale
behind this suggestion was that HULIS in aerosol were thought
to be chemically indistinguishable from the wide variety of
natural humic substances present in other reservoirs.
Using the same method Subbalakshmi et
al. found similar
compounds in urban aerosol, except that there higher substituted
lignin pyrolysis products were also observed (2001). The pyrograms
of biomass burning aerosol from Brazil, however, revealed some
differences with respect to those of rural fine aerosol (Blazsó
et al., 2003). Most
importantly, in biomass burning aerosol there were several higher
substituted aromatic compounds which were absent from rural
aerosol. These species—which are typical lignin degradation
products—were also shown to be present in the pyrogram of soil
humic and fulvic acids (Martin et al., 1994). This finding made Gelencsér et al. reconsider their previous results on HULIS in rural fine aerosol
(Gelencsér et al.,
2002). Their conclusion was that in spite of all apparent similarities,
these differences unambiguously prove the disparate origin of
HULIS, namely their atmospheric formation in heterogeneous or
multiphase processes.
back to top
Laboratory evidence for the formation of HULIS in heterogeneous and multiphase
processes
Several hypothesis that heterogeneous
or multiphase reactions can lead to the formation of humic-like
substances (HULIS) as major SOA components were put forward
by groups in Europe and the US. Gelencsér et
al. (2002) observed HULIS formation in liquid phase from
organic acids which are ubiquitous in the atmosphere. Jang et
al. (2002) have presented laboratory evidence on acid-catalyzed
heterogeneous carbonyl chemistry on aerosol particles, including
various acid-catalyzed reactions, such as hydration, hemiacetal
and acetal formation, aldol condensation, and polymerization
in the aerosol phase. In terms of possible HULIS formation it
is important that equilibrium between an aldehyde and its hydrate
favors the hydrate form and reacts further with carbonyls to
yield dimers, trimers, and polymers. However, it is important
to point out that these reactions, even polymerization are thought
to be reversible.
Limbeck et al. (2003) have then presented
laboratory evidence for the irreversible formation of HULIS
in heterogeneous reactions for the case of dienes like isoprene
in the presence of sulfuric acid. The reactions yielded colored
polymeric products whose humic-like character was evidenced
by UV-spectrometry, thermal analysis, and FTIR diffuse reflectance
spectroscopy. The authors hypothesized that isoprene—whose SOA
formation was thought to be negligible (Pandis et al., 1991, Griffin et al., 1999)—is
processed to humic-like polymers (HULIS) on highly acidic atmospheric
sulfate clusters. It should be noted that these experiments
were performed in bulk.
In the same year, Gelencsér et
al. (2003) presented
evidence about the irreversible formation of HULIS in atmospheric
multiphase reactions in the laboratory. The precursors were
aromatic hydroxy acids which are abundant lignin pyrolysis products
in biomass burning aerosol. They showed that even a single representative
compound can react with OH radicals yielding colored products
under typical conditions prevalent in cloud water. The time-scale
of the reactions was found to be hours, implying that the process
does have atmospheric significance. The reactions proceed by
radical dimerization and oligomerization to yield higher molecular
weight products. A follow-up study on the molecular weights
of the reaction products has revealed a continuous distribution
well below 1000 Dalton, which confirms the high degree of similarity
to atmospheric HULIS (Hoffer et
al., 2004). In addition, this study has proven conclusively
that the process is oligomerization rather than polymerization.
In the same study, the results implied that HULIS consist of
condensed and partially oxidized (e.g. quinone-like) phenolic
structures crosslinked with short-chain aliphatic bridges which
form by the oxidative cleavage of the phenolic ring.
Two smog-chamber studies
on the formation of HULIS or oligomers have been reported until
now in 2004.
Iinuma et
al., (2004) investigated the ozonolysis of alpha-pinene in the
presence of acidic particles. A
thermographic method for the determination of TOC showed an
increase of particle phase organics by 40% for the experiments
with higher acidity. CE-ESI-MS analysis showed a large increase
in the concentration of compounds with Mw>300
from the experiments with sulfuric acid seed particles. Although
in this paper the term HULIS is not used, the apparent similarity
of the oligomers to HULIS (or vice
versa) in their molecular weight distribution may entitle
us to use these terms interchangeably.
Kalberer
et al. (2004) showed recently, that atmospheric polymers may
form also from light initiated photochemistry of a trimethylbenzene
– NOx mixture.
Experimental and/or observational evidences back up all the
hypothesis presented here, and so far there have been three
studies in smog chambers which all support the oligomer formation.
It is possible or even likely that to a certain extent all mechanisms
could be operative — this would explain the ubiquitous nature
and abundance of HULIS in continental fine aerosol. What is
known for certainty is that biomass burning is a source of HULIS—however
its secondary origin from biogenic as well as anthropogenic
sources, and in particular the aerosol formation rates, is still
to debated.
Figure
1: Pathways of HULIS formation
5. Organic Carbon Mass Balance
A remarkable set of new organic species of oxygenated organic
compounds as well as of the group parameter “humic like substances”
have been identified in the last years in the atmospheric aerosol.
However, still there is a lack of quantitative data for different
environments. Available data expressed as % of “compound-carbon” from OC
are compiled in Table 2. The
largest contribution of an individual group of compounds stems
from humic like substances, which occur in the atmospheric aerosol
in water soluble and water insoluble forms.
The group of “saccharides” include levoglucosan, which
is a tracer for wood or other biomass combustion. Based on test
fires with different types of log wood a relation of 100+/-40
mg of Levoglucosan per gram of fine particle emitted OC was
derived (Fine et al. 2001). Thus, from Levoglucosan levels in
the aerosol the biomass-OC fraction can be estimated from Biomass-OC
= 10* Levoglucosan (Eq. 1). Organic aerosol levels as derived
from Levoglucosan data range from 5-50% at European as well
as Tropical sites. OC from biomass combustion, however contains
humic like substances. Therefore it is not possible at present
to sum up the group contributions from biomass burning as derived
from equation 1 and the group of HULIS.
Table 2: Potential for contribution of different organic species
or groups to % of OC observed at US or European Continental
sites
|
%C of OC |
|
Reference |
Alkanes - C |
1 |
|
|
Unresolved Complex Mixture-C |
5 |
|
|
Mono- and dicarboxylic
acids-C |
5-10 |
|
|
Tricarboxylic acids |
? |
Amazonia 6%
|
Zdrahal et al.
2001 |
Tetrols - C |
? |
Amazonia 10-20%
|
Claeys et al.
2004 |
Plant Debris (Cellulose)-C |
1-5 |
Vienna |
Puxbaum &
Tenze-Kunit 2003 |
Bacteria & Spores
- C |
1-5 |
Austria/Alpine |
Bauer et al.
2003b |
“Saccharides” - C
|
0,3-2 |
Ghent
Up to 5% Rhondonia |
Zdrahal et al.
2002 |
HULIS
Water soluble-C
|
7-24 |
|
|
HULIS Water insoluble-C |
5-20 |
|
|
Sum |
20-80 |
|
|
6.
Recommendations
References
Bauer
H., Kasper-Giebl A., Zibuschka F., Kraus G.F., Hitzenberger
R., Puxbaum
H. (2002a) Determination of the carbon content
of airborne fungal spores. Anal. Chem. 74,
91-95.
Bauer H., Kasper-Giebl A., Löflund M., Giebl H., Hitzenberger R., Zibuschka
F., Puxbaum
H. (2002b) The contribution of
bacteria and fungal spores to the organic carbon content
of cloud water, precipitation and aerosols. Atmos. Res.
64, 109-119.
Bauer H., Giebl H., Hitzenberger R., Kasper-Giebl A., Reischl G., Zibuschka F., Puxbaum H. (2003) Airborne bacteria as cloud
condensation nuclei. J. Geophys. Res. 108, D21, 4658, 10.1029/2003JD003545.
Blazsó M., Janitsek S., Gelencsér A., Artaxo
P., Graham B., Andreae M. (2003) Study of tropical organic aerosol
by thermally assisted alkylation-gas chromatography mass spectrometry.
J. Anal. Appl. Pyrolysis 68-69, 351-369.
Claeys M., Graham B., Vas G., Wang W., Vermeylen
R., Pashynska V., Cafmeyer J., Guyon P., Andreae M.O., Artaxo
P., Maenhaut W. (2004) Formation of secondary organic aerosol
through photooxidation of isoprene. Science 303, 1173-1176.
Decesari
S, Facchini MC, Fuzzi S, et al. Characterization of water-soluble
organic compounds in atmospheric aerosol: A new approach. J.
Geophys. Res. 105 (D1), 1481-1489, 2000.
Decesari
S, Facchini MC, Matta E, Lettini F, Mircea M, Fuzzi S, Tagliavini
E, Putaud JP. Chemical features and seasonal variation of fine
aerosol water-soluble organic compounds in the Po Valley, Italy,
Atmos. Environ. 2001,
35, 3691-3699.
Ellis,
E. C., and T. Novakov: Application of thermal-analysis to the
characterization of organic aerosol particles. Sci.
Tot. Environ. 23, 227-238, 1982.
Facchini, M.C., Fuzzi, S., Zappoli,
S., Andracchio, A., Gelencser, A., Kiss, G., Krivacsy, Z., Meszaros,
E., Hansson, H.-C., Alsberg, T., Zebuhr, Y., 1999.
Partitioning of the organic aerosol component
between fog droplets and interstitial air.
Journal of Geophysical Research, 104,
26821-26832.
Fuzzi
S, Decesari S, Facchini MC, et al. A simplified model of the
water-soluble organic component of atmospheric aerosols, Geophys.
Res. Lett. 28 (21), 4079-4082, 2001.
Gelencsér A, A. Hoffer, G. Kiss, E. Tombácz,
R. Kurdi, L. Bencze, In-situ formation of light-absorbing organic
matter in cloud water,
J. Atmos. Chem.
45, 25-33, 2003.
Gelencsér,
A., A. Hoffer, Z. Krivácsy, G. Kiss, A. Molnár, and E. Mészáros,
On the possible origin of humic matter in fine continental aerosol.
J. Geophys. Res. 107, D21,
doi: 10.1029/2001JD001299, 2002.
Gelencsér,
A., Hoffer, A., Molnár, A., Krivácsy, Z., Kiss, G., Mészáros,
E.: Thermal behaviour of carbonaceous aerosol from a continental
background site. Atmos. Environ. 2000a, 34, 823-831.
Gelencsér,
A., Sallai, M., Krivácsy, Z., Kiss, G., Mészáros, E.:
Voltammetric evidence for the presence of humic-like substances
in fog water. Atmos. Res. 2000b, 54, 157-165.
Gelencsér, A., T. Mészáros, M. Blazsó, G. Kiss, Z. Krivácsy,
A. Molnár, E. Mészáros, Structural characterisation of organic
matter in fine tropospheric aerosol by pyrolysis-gas chromatography-mass
spectrometry, J. Atmos. Chem., 2000c, 37, 173-183.
Graham, B.; Guyon, P.; Taylor,
P.E.; Artaxo, P.; Maenhaut, W.; Glovsky, M.M. ; Flagan, R.C.;
Andreae, M.O. (2003) Organic compounds present in the natural
Amazonian aerosol: characterization by gas chromatography-mass
spectrometry. Journal of Geophysical Research, [Atmospheres]
(2003), 108(D24), AAC 6/1-AAC 6/13. CODEN: JGRDE3 ISSN:0148-0227.
AN 2004:193006
Griffin, R. J., Cocker III, D. R.,
Flagan, R. C. & Seinfeld, J. H. Organic aerosol formation
from the oxidation of biogenic hydrocarbons. J.
Geophys. Res. 104,
3555-3567 (1999).
Havers,
N., Burba P., Lambert, J., Klockow, D., 1998. Spectroscopic
characterisation of humic-like substances in airborne particulate
matter. Journal of Atmospheric Chemistry 29, 45-54.
Hoffer, A., G. Kiss, M. Blazsó, A. Gelencsér, Chemical
characterization of humic-like substances (HULIS) formed from
a lignin-type precursor in model cloud water, Geophys.
Res. Lett., in press.
Iinuma
Y., Boge O., Gnauk T., Herrman H. (2004) Aerosol-chamber study
of the a-pinene/O3 reaction: influence of particle acidity on
aerosol yields and products Atmospheric
Environment 38, 761-773.
Jang,
M., Czoschke N., Lee S., Kamens, R. M. (2002) Heterogeneous
atmospheric aerosol formation by acid catalyed particle-phase
reactions. Science 298, 814-817
Jang, M., Kamens, R. (2001) Atmospheric
secondary aerosol formation by heterogeneous reaction of aldehydes
in the presence of a sulfuric acid aerosol catalyst. Environ.
Sci. Technol.
35, 4758-4766 (2001).
M.
Kalberer, D. Paulsen, M. Sax, M. Steinbacher, J. Dommen, A.S.H.
Prevot, R. Fisseha, E.Weingartner, V. Frankevich, R. Zenobi,
and U. Baltensperger (2004) Identification of Polymers as Major
Components of Atmospheric Organic Aerosols. Science 303, 1659-1662.
Kavouras, I. G., Mihalopoulos, N. &
Stephanou E. G. Formation of atmospheric particles from organic
acids produced by forests. Nature 395, 683-686 (1998).
Kiss,
G., B. Varga, I. Galambos, I. Ganszky, Characterization of water-soluble
organic matter isolated from atmospheric fine aerosol, J. Geophys. Res, 107, D21, 8339, doi.: 10.1029/2001JD000603,
2002.
Kiss,
G., E. Tombácz, B. Varga, T. Alsberg, L. Persson, Estimation
of the average molecular weight of humic-like substances isolated
from fine atmospheric aerosol, Atmos. Environ. 37, 3783-3794, 2003.
Krivácsy,
Z., Kiss, G., Varga, B., Galambos, I., Sárvári, Zs., Gelencsér,
A., Molnár, A., Fuzzi,
S., Facchini, M.C., Zappoli, S., Andracchio, A., Alsberg, T.,
Hansson, H.-C., Persson, L.: Study of humic-like substances
in fog and interstitial aerosol by size-exclusion chromatography
and capillary electrophoresis. Atmos. Environ. 2000, 34, 4273-4281.
Kunit,
M., Puxbaum H., 1996. Enzymatic determination of the cellulose
content of atmospheric aerosols. Atmospheric Environment 30, 1233-1236.
Limbeck
A., Kulmala M., Puxbaum H. (2003) Secondary organic aerosol
formation in the atmosphere via heterogeneous reaction of gaseous
isoprene on acidic particles. Geophysical Research Letters 30(19), 1996, doi:10.1029/2003GL017738.
Martin,
F., F. J. González-Vila, J. C. del Rio, and T. Verdejo, Pyrolysis
derivatization of humic substances 1. Pyrolysis of fulvic acids
in the presence of tetramethylammonium hydroxide, J. Anal. Appl. Pyrol., 28, 71-80, 1994.
Matthias-Maser,
S., Jaenicke, R., 1995. The size distribution of primary
biological aerosol particles with radii > 0.2 µm in an urban/rural
influenced region. Atmospheric Research 39, 279-286.
McCrone
W.C., Delly J.G., 1973. The Particle Atlas (Ed. 2) Volumes I-IV,
Ann Arbor Science Publishers, Ann Arbor, Michigan.
Mukai, H. & Ambe, Y. Characterization
of a humic acid-like brown substance in airborne particulate
matter and tentative identification of its origin. Atmos.
Environ. 20, 813-819 (1986).
Pandis
S.N., S. E. Paulson, J. H. Seinfeld, and R. C. Flagan, Aerosol
formation in the photooxidation of isoprene and b-pinene,
Atmos. Environ. 25A,
997-1008, 1991.
Puxbaum
H. (1979) Thermo-Gasanalysator zur Charakterisierung von Kohlenstoff-
und Schwefelverbindungen in luftgetragenen Stäuben. Fresenius
Zeitschrift für Analytische Chemie 298, 250-259.
Puxbaum
H., Rendl J., Allabashi R., Otter L., and Scholes M.
C. (2000) Mass balance of atmospheric aerosol in a South-African
subtropical savanna (Nylsvley, May 1997). J. Geophys. Res. 105,
20697-20706.
Puxbaum,
H., Tenze-Kunit, M. (2003) Size distribution and seasonal variation
of atmospheric cellulose. Atmospheric Environment 37, 3693-3699.
Rogge,
W. F., Mazurek, M. A., Hildemann, L. M., Cass, G. R., Simoneit,
B. R. T . (1993a) Quantification of urban organic aerosols at
a molecular level: identification, abundance and seasonal variation.
Atmos. Environ. 27A,
1309-1330.
Rogge,
W. F., Hildemann, L. M., Mazurek, M. A., Cass, G. R., Simoneit,
B. R. T., 1993b. Sources of fine organic aerosol, 4. Particulate
abrasion products from leaf surfaces of plants. Environmental
Science and Technology 27, 2700-2711.
Sattler
B., Puxbaum H., and Psenner R. (2001) Bacterial growth in supercooled
cloud droplets. Geophys. Res. Lett. 28/2, 239-242.
Saxena, P. & Hildemann, L.M. Water-soluble
organics in atmospheric particles: A critical review of the
literature and application of thermodynamics to identify candidate
compounds. J. Atmos. Chem. 24, 57-109 (1996).
Schäppi, G.F., Taylor, P.E., Staff,
I.A., Suphioglu, C., Knox, R.B. (1997) Source of Bet v 1 loaded
inhalable particles from birch revealed. Sex Plant Reprod 10,
315-323.
Simoneit B. (1980) Eolian particulates
from oceanic and rural areas – their lipids, fulvic and humic
acids and residual carbon. Physics and Chemistry of the Earth,
12 (Adv. Org. Geochem.) 343-352.
Simoneit,
B. R. T., and M. A. Mazurek, Organic matter of the troposphere,
II. Natural background of biogenic lipid matter in aerosols
over the rural western United States, Atmos. Environ., 16, 2139-215, 1982.
Simoneit,
B. R. T., Organic matter in eolian dusts over the Atlantic Ocean,
Mar. Chem., 5, 443-464, 1977.
Simoneit,
B.R.T, Schauer, J.J., Nolte, C.G., Oros, D.R., Elias, V.O.,
Fraser, M.P., Rogge, W.F., Cass G.R. (1999) Levoglucosan, a
tracer for cellulose in biomass burning and atmospheric particles.
Atmospheric Environment 33, 173-182.
Subbalakshmi,
Y., A. F. Patti, G. S. H. Lee, and M. A. Hooper, Structural
characterisation of macromolecular organic material in air particulate
matter using Py-GC-MS and solid state 13C-NMR, J. Environ. Monit. 2, 561-565, 2000.
Varga,
B., Kiss, G., Ganszky, I. Gelencsér, A., and Krivácsy, Z.: Isolation
of water soluble organic matter from atmospheric aerosol. Talanta
2001, 55, 561-572.
Weissenbök
R.H., Currie L.A., Gröllert C., Kutschera W., Marolf
J., Priller A., Puxbaum H., Rom W., Steier P. (2000)
Accelerator mass spectrometry analysis of non-soluble carbon
in aerosol particles from high alpine snow (Mt. Sonnblick, Austria).
Radiocarbon 42, 285-294.
Yu,
J. Z., H. Yang, H. Y. Zhang, A. K. H. Lau, Size distributions of water-soluble organic
carbon in ambient aerosols and its size-resolved thermal characteristics,
Atmos. Environ. 38 (7), 1061-1071,
2004.
Zhang Q., Anastasio C. (2003) Free
and combined amino compounds in atmospheric fine particles (PM2.5)
and fog waters from Northern California. Atmospheric Environment
36, 2247-2258.
Zappoli,
S., Andracchio, A., Fuzzi, S., Facchini, M.C., Gelencser, A.,
Kiss, G., Krivacsy, Z., Molnar, A., Meszaros, E., Hansson, H.-C.,
Rosman, K., Zebühr, Y., 1999. Inorganic, organic and macromolecular
components of fine aerosol in different areas of Europe in relation
to their water solubility. Atmospheric Environment 33, 2733-2743.
Zdrahal
Z., Vermeylen R., Claeys M., Maenhaut W., Guyon P., Artaxo P.
(2001) Characterisation of novel di- and tricarboxylic acids
in fine tropical aerosols. Journal of Mass Spectrometry 36,
403-416.
Zdrahal
Z., Oliveira J., Vermeylen R., Claeys M., Maenhaut W. (2002)
Improved method for quantifying Levoglucosan and related monosaccharides
in atmospheric aerosols and application to samples from urban
and tropical locations. Environmental Science and Technology
36, 747-753.
back
to top