Topic Leader: Lara A. Gundel
Contributors: Douglas A. Lane, John Volcken
Abstract
1a.
How are SVOC and PM-associated OC defined?
i.
Theoretical Definitions
SVOC
Borrowing from Van Vaeck et al (1984) and others (for example,
Finlayson-Pitts and Pitts, 2000, p 412) we define semi-volatile
organic compounds (SVOCs) as organic compounds that show significant
gas and particulate concentrations in the atmosphere. Pankow
(1993) generalized this by including other surfaces. He observed
that SVOCs can have non-negligible fractions of their environmental
masses in both the atmosphere and partitioned to surfaces such
as soil, plants, building materials and sampling media. SVOCs
have vapor pressures between about 10-4 or 10-5 and 10-11 atm
(100.1 and 10-6 Pa; 10-1 and 10-8 Torr) over the ambient temperature
range. Compounds with higher vapor pressures are present primarily
in the gas phase, whereas low volatility compounds are found
on or within particles.
SVOCs are ‘sticky’ or multi-phasic; they partition
their mass between the gas phase and any surfaces that afford
a degree of sorption, such as fine particles in air or sampling
media. The degree of gas-particle partitioning affects the transport,
deposition, and atmospheric fate of these compounds, since SVOCs,
once airborne, can deposit onto vegetative surfaces, windows,
carpets, soils, or even the human body.
Nearly all classes of organic compounds contain semi-volatiles:
alkanes, PAHs, PCBs, PCDDs, PBDEs, nitro-aromatics, terpenes,
acids, carbonyls, and lipids, to name a few. SVOCs enter the
atmosphere by direct emission, frequently as byproducts of incomplete
combustion. Polar SVOCs are also produced by oxidation of precursor
unsaturated compounds, and they can be incorporated into PM
as secondary organic aerosols (SOA). Precursor organics are
emitted from transportation, industrial and biogenic sources.
Also, many of the potentially carcinogenic organic compounds
found in the atmosphere are semi-volatile.
PM-associated OC
Roughly half the mass of urban fine particles in the US can
be attributed to carbonaceous components. From 10 to 30% of
this is elemental or black carbon, and carbonate-containing
compounds have negligible contributions. Here we define PM-associated
organic carbon as the complex mixture of organic compounds that
are incorporated into airborne particles by direct emission,
abrasion, condensation and surface reactions. The term PM-associated
OC may be useful when considering the influence of the complex
mixture of particle-associated organic compounds as a whole,
for example, when investigating aerosol properties like organic
film thickness, hydrophilicity, and optical absorption.
Relationships between SVOCs and PM-associated OC:
gas/particle partitioning
SVOCs and PM-associated OC are related through the partitioning
of SVOCs onto PM. However, less than half of PM-associated OC
is semi-volatile under temperate conditions. The partition coefficient
Kp is the most commonly used parameter for describing gas/particle
partitioning, primarily because of its log-linear relationship
to compound vapor pressure, poL. Although a compound’s
vapor pressure at the temperature of interest has the greatest
influence on partitioning, the interaction between compound
structure and the sorptive medium plays an important role (e.g.,
compound size and polarity vs. adsorptive affinity or absorptive
capacity). Plots of log Kp vs. log poL can provide information
on the nature of the partitioning and may indicate whether sampling
artifacts have impacted the measurement. Gas/filter partitioning
coefficients can also be used to improve sampler and field study
design (Mader et al., 2001).
Using Pankow’s nomenclature (as presented in Ch. 3 of
Lane, ed. 1999), the equilibrium partitioning of a semi-volatile
compound to an environmental surface S can be represented most
simply by
G + S = P (1)
where G and P represent the gas and particulate phases of the
SVOC. Since the gas and particulate phase concentrations are
usually collected on an adsorbent and filter, respectively,
their concentrations have been conveniently represented by A
and F in the literature. If the sorbing surface is total suspended
particulate matter, its concentration can be represented by
TSP. (G/P theory takes the same form for size segregated particles,
but TSP is used here to be consistent with Pankow’s development.)
At equilibrium the gas/particle partitioning constant Kp for
adsorption of the SVOC compound i onto the solid surface of
a particle can be expressed as in (2):
Adsorption to a solid surface (2)
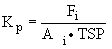
Pankow (1987) showed that Langmuir adsorption theory predicts
that Kp (at constant temperature) is inversely proportional
to the vapor pressure of i. If i is a solid, the sub-cooled
liquid vapor pressure poL is used.
adsorption (3)
Ns and atsp are terms for the number of adsorption sites per
unit area and the surface area of the particles, respectively.
For compounds of the same class, with similar enthalpies of
desorption and vaporization Q among the members, plots of log
Kp versus log poL for will be linear slope of –1, as shown
in (4).
adsorption (4)
SVOCs can also absorb into liquid particles such as environmental
tobacco smoke or liquid (organic and/or water) films on particles
with solid cores, as Pankow and colleagues have shown. Gas/particle
partitioning of SVOCs in urban areas is better explained as
absorption than adsorption. The absorptive partitioning of SVOC
i into a liquid organic layer on a particle is like a gas dissolving
in a liquid (Finlayson-Pitts and Pitts, 2000, p 417), and the
measured partitioning coefficient for absorption takes the same
form as (2).
absorption into a liquid film or droplet (5)
Fi,om represents the particle- associated concentration of
i in air as measured from a filter, with explicit recognition
that i has dissolved in liquid organic material, om, on the
particle. For absorption into liquid films on particles, Pankow
(1994) showed that Kp is proportional to the weight fraction
of om to TSP.
adsorption (6)
Kp is inversely proportional to the product of poL and the
activity coefficient g of i in the liquid phase. Vapor pressure
is the most important factor influencing Kp, followed by activity
coefficient and molecular weight. If the activity coefficient
does not vary much across members of a class of SVOCs, plots
of Kp vs log poL will have a slope of -1 for both adsorption
and absorption, as in equations (4) and (7).
(7)
Recent contributions to gas/surface partitioning theory address
observed deviations from the predictions of equations (4) and
(7). Jang, Kamens et al. (1997) applied a comprehensive thermodynamic
approach to calculate group contributions to activity coefficients
for adsorption of SVOC into non-ideal organic films. This allows
calculation of activity-normalized partitioning coefficients,
Kp,g. Goss and Schwarzenbach (1998) argued that slope deviations
from –1 in log Kp vs log poL plots do not necessarily
indicate non-equilibrium conditions, and they indicated how
these deviations can be used to identify types of sorbate/sorbent
interactions and thus characterize sorption processes. For example,
they showed how acid/base interactions can influence gas/surface
partitioning polar SVOC. Harner and Bidleman (1998) demonstrated
that using laboratory-derived octanol/air partitioning coefficients
circumvents the need to estimate activity coefficients for compounds
absorbed in the organic films that coat urban particles. Mader
et al. (2000, 2001) expanded partitioning theory to quartz and
Teflon filter materials that are used to collect particles,
thus tackling the sticky problem of SVOC adsorption artifacts
in PM sampling on filters.
ii.
Operational Definitions
Partitioning constants are calculated from measured concentrations
of individual compounds in each phase. The terms Ai and Fi in
equation (2) must be determined for individual compounds. For
some classes of compounds, good estimates of vapor pressures
can be calculated from observed molecular weight-vapor pressure
relationships for members of the class. Whereas concentrations
of PM-associated OC can be determined experimentally as the
collective concentration of ‘organic carbon’ by
programmed evolved gas analysis, at present there is no way
to determine total airborne concentrations of ‘semi-volatile
organic carbon’ in an analogous way. PM-associated OC
includes temperature dependent PM-associated SVOC of largely
uncertain composition. Thus, at present it is not possible to
distinguish SVOC and PM-associated OC quantitatively and accurately
without introducing technique-dependent bias.
As Turpin et al. (2000) point out, the term SVOC is usually
operationally defined, or undefined, as below:
=
concentration of i measured from filter F, and (8a)
=
concentration of I measured from adsorbent A (8b)
Because the typical meaning of SVOC is rooted in sampling strategy,
Turpin et al. (2000) prefer to use the term ‘condensable’
for airborne compounds that are found in both the gas and particulate
phases.
The total carbon content of PM-associated OC can be determined
by thermal analysis, as discussed at the OC/EC workshop in 2003.
At present, measured concentrations of PM-associated OC depend
on both the sampling and analytical methods. Assuming negligible
particulate carbonate,
Particulate C = PM-assoc. organic carbon (OC) + elemental carbon
(EC). (9)
However, even the widely used thermal-optical differentiation
of PM-assoc. organic carbon from elemental carbon depends on
operationally defined algorithms. If i and j represent individual
semi-volatile and non-volatile organic species,
(10)
At present, the SVOC term in equation (10) can be estimated
from thermal methods for particulate C (Fan et al., 2003) and
possibly from source characterization (Schauer et al.), but
accurate determination of the organic carbon content of real-world
SVOC (as a class) remains elusive.
Fig. 1 has schematic representations of air sampler configurations
that lead to different operational definitions of particulate-associated
organic carbon. Component descriptors are given on the left,
while the labels on the right indicate the function of each
section. F denotes a particle-collecting section (usually, but
not always a filter; sometimes a sorbent that collects SVOC
evaporating from the particles during sampling). A stands for
the section that collects gas phase SVOC (sorbent-coated denuder,
sorbent such as XAD resin, or backup filter for estimating the
gas phase SVOC adsorption artifact that occurred on F).
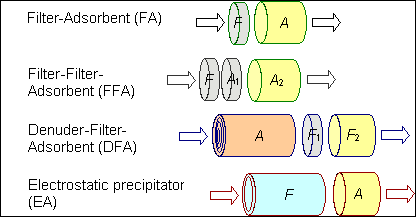
Figure 1. Sampler
designs with different operational definitions of particulate-associated
organic carbon. The arrows show the direction of flow. The usual
component descriptors are shown on the left, but the descriptors
may not accurately describe the original phase of airborne SVOC
that collect there. Origins are indicated using the notation
of equation (8).
Below are the operational definitions of the gas and particulate
concentrations of SVOCi for the sampler designs in Fig 1.
(FA, DFA and EA) [SVOCi]g = Ai (11a)
(FFA) [SVOCi]g = A1i + A2i (11b)
(FA and EA) [SVOCi]p = Fi (12a)
(FFA) [SVOCi]p = Fi - A1i (12b)
(DFA) [SVOCi]p = F1i + F2i (12c)
Partitioning coefficients derived from these designs differ,
as discussed in Topic 1c.
1b.
What are the potential bias or problems associated with different
PM and SVOC sampling techniques?
Filter-Adsorbent (FA) with configuration FA; equations (11a)
and (12a).
This is often referred to as the ‘conventional’
sampler design, since it has been used for at least two decades
for speciation of semi-volatile and particulate organics in
airborne PM. The sorbent A is typically polyurethane foam, XAD
resin or a foam/XAD/foam sandwich.
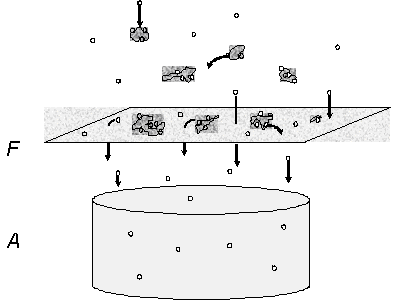
Figure 2. Filter-Adsorbent
or conventional sampling configuration for sampling airborne
semi-volatile and particulate organic compounds.
At equilibrium SVOC adsorb and desorb from particles. The
upper part of Figure 2 shows adsorbed SVOC as small dots associated
with the irregularly shaped particles. Particulate SVOC are
trapped on the filter medium F, and gases pass through the filter
F for adsorption on A. Positive sampling artifacts occur when
gaseous SVOC adsorb to the filter medium, as shown by small
dots on the filter. Negative artifacts result from particulate
SVOC evaporating from the filter deposit during sampling. The
volatilized SVOC become trapped on the adsorbent A.
McDow and Huntzicker (1990) found that sampling artifacts for
OC depend on face velocity and sampling duration. Turpin et
al. (1994) showed that the quartz filter positive artifact decreased
as a fraction of the total OC, with increasing sampling time,
at constant face velocity and particle mass concentration. After
reviewing studies available at the time, Turpin et al. (2000)
concluded that positive artifacts for OC are usually larger
than negative artifacts when sampling urban air. Gas/particle
partitioning measurements of individual semivolatile compounds
typically indicate that negative artifacts dominate (Van Vaeck
et al., 1984; polycyclic aromatic hydrocarbons (Fan et al.,
19xx); a variety combustion-generated organics, mostly non-polar
or moderately polar species, Schauer et al., 19xx, Schauer et
al., 19xx).
Filter-Filter Adsorbent (FFA) with configuration FAA;equations
(11b) and (12b)
Two filters in series are often used as a simple sampling approach
to correct for positive artifacts in sampling particulate-associated
OC. The FFA design with configuration FAA has not been used
widely for gas/particle partitioning of individual species.
Assuming that the upstream filter has collected a negligible
fraction of the total airborne SVOC, the downstream filter,
used as the adsorbent A1 for SVOCg, has the close to same amount
of adsorbed SVOC as the upstream, particle-laden filter. The
remainder of the airborne SVOC will be trapped if an adsorbent
component A2 is used.
An improvement is to use co-located filter pairs. The upstream
filters are Teflon and quartz, but both downstream filters are
quartz. This approach is sometimes called tandem sampling with
the pairs referred to as TQ and QQ. The OC determined from the
quartz behind Teflon is subtracted from the OC on the upstream
quartz filter. This should represent the positive artifact more
accurately, since Teflon filters do not have the adsorptive
capacity of quartz. Turpin et al. (2000) concluded that artifact
OC measured from A1 could be up to 40% higher if determined
from the quartz behind Teflon rather than quartz behind quartz.
Denuder-Filter-Adsorbent (DFA) with configuration AFF; equations
(11a) and (12c)
When gas phase SVOC are collected upstream of filters, positive
artifacts can be minimized. This process denudes the gas stream
of its SVOC, and the adsorption occurs in a denuder. Because
the gas/particle partitioning equilibrium is disturbed during
sampling, particulate SVOC become more susceptible to volatilization.
A post-filter adsorbent, F2 in Fig. 1, traps semivolatile species
that have evaporated from particles on F1.
How denuders work: DL slide 5
Lane et al. (198x, reviewed in chapter x of Lane, 1999) and
Eatough et al. (199x) pioneered application of denuder difference
methods for sampling airborne semi-volatile and particulate
organics. To remove SVOC upstream of filters, Lane et al. used
Tenax-impregnated GC-stationary phase gum on glass annular denuders,
while Eatough et al. use parallel strips of activated carbon-impregnated
paper. Sorbents (F2) were used downstream of the filters. These
designs require co-located conventional samplers (FA) for gas/particle
partitioning measurements because SVOC can not be determined
from the denuders. Gundel et al. (1995) introduced extractable
XAD-coated denuders for direct determination of gas and particle
concentrations without the need for co-located conventional
samplers. XAD-coated denuders and filters have been incorporated
into several sampler designs (Gundel et al., 1999; Lewtas et
al., 2001; Mader et al., 2001c; Hammond et al., 2003).
So far, the DFA (AFF) sampling approach has worked better for
gas/particle partitioning of individual species than for determination
of aggregate particulate SVOC as OC.
Electrostatic precipitator (EA) with configuration FA
Dr. Douglas Lane will discuss how characterization of particle-associated
organic species can be affected by the sampler geometry. Collected
PM should be representative of the PM composition at the time
of sampling, and not influenced by volatilization of SVOCs from
the collected particles or sorption of SVOCs to the particles
or sampling media. From the perspective of particle composition,
these processes lead to negative and positive sampling artifacts,
respectively, and they will be illustrated for samplers that
collect particles onto filters upstream of sorbents for SVOC.
Multi-channel annular diffusion denuders are being used to minimize
positive PM artifacts. They have also been incorporated upstream
of filters in the filter/sorbent geometry for determination
of the gas/particle partitioning coefficients of source and
atmospheric SVOCs. Data from recent field measurements using
the Integrated Organic Gas and Particle Sampler (IOGAPS) will
be presented to demonstrate the uses and limitations of denuder-based
samplers. The application of denuders in smog chamber studies
will be illustrated with gas/particle partitioning of the products
of reactions of PAH with OH. The importance of understanding
the limitations of air samplers and the proper selection of
a sampler for a particular sampling objective will be emphasized.
1c. Advances in sampling and analysis of SVOC
Dr. John Volckens will 1) highlight recent advances in sampling
and analysis of SVOCs, 2) discuss techniques to interpret artifact-biased
data, and 3) recommend strategies for future research based
on needs of the community.
As mentioned in section 1a, the ratio Kp is the most commonly
used parameter for describing gas-particle partitioning, primarily
because of its log-linear relationship to compound vapor pressure,
poL. Gas-particle partitioning ratios (Kp) are defined at equilibrium,
when the rates of mass transfer between phases are equal and
at steady state. However, the conditions governing SVOC equilibrium
are easily disrupted, especially when trying to measure gas-particle
phase distributions in air. However, care must be taken with
the use of Kp because this ratio is easily corrupted by even
minute artifacts. Such sampling artifacts are widely known but
difficult to prevent, predict, or account for after the fact
(Volckens and Leith, 2003).
Plots of log Kp vs. log poL can provide information on the
nature of the partitioning and may indicate whether sampling
artifacts have impacted the measurement. Furthermore, most time-integrated
sampling techniques (i.e. filter) cannot provide a true representation
of ‘average Kp’. A need exists to develop improved
sampling techniques with less perturbation of semi-volatile
equilibrium, shorter sampling periods, and lower limits of detection.
References: